Technology to support the decarbonisation of HGVs
Abbreviations
The following is a list of abbreviations and associated definitions for terms appearing throughout this document:
- BET - Battery Electric Truck
- Bio- (as a prefix, e.g. to “LNG”) - A type of the prefixed fuel that is derived from organic matter (biomass), not fossil sources.
- CET - Catenary Electric Truck
- CNG - Compressed Natural Gas
- EU - European Union
- RS - Electric Road System
- FCET - (Hydrogen) Fuel Cell Electric Truck
- Fuel Cell - A device that reacts stored hydrogen with oxygen from the air to provide electrical power
- GHG - Greenhouse Gases
- ICE - Internal Combustion Engine
- HDV - Heavy Duty vehicle – road vehicle over 3.5T, e.g. HGV, buses, coaches and ‘vocational’ vehicles such as gritters, refuse collection vehicles
- HGV - Heavy Goods Vehicle
- LCF - Low Carbon Fuels
- LNG - Liquefied Natural Gas
- OEM - Original Equipment Manufacturers
- RTFO - Renewable Transport Fuel Obligation
- R&D - Research and Development
- SME - Small and Medium Enterprises
- TCO - Total Cost of Ownership
- UK - United Kingdom
- ULEV - Ultra Low Emission Vehicle
- ZETs - Zero Emission Trucks
Introduction
Purpose of this paper
The purpose of this paper is to develop a common level of understanding amongst Taskforce members of:
- Technologies expected to contribute to the pathway to zero emission trucks
- The state of play of these technologies in Scotland and globally, including availability, limitations in use and dependencies
- A comparison of how these technologies may be relevant to the HGVs in Scotland (as described in Background Paper 1, Industry overview and SWOT)
Definitions and Assumptions
Zero-emission HGVs are defined as HGVs that have zero tailpipe (pump to wheel) greenhouse gas emissions at point of use. Embodied emissions associated with the creation, maintenance and disposal of vehicles or infrastructure and production and distribution of energy (well to pump) are recognised as being important but are outside the scope of the Taskforce.
This Paper focuses on technology solutions expected to make significant contributions to the pathway to zero emission trucks through reducing tailpipe emissions. It does not address technology solutions associated with operational changes such as platooning, smarter logistics, fleet design, efficient driving techniques or co-loading.
Policy Context
A full policy context is provided in Background Paper 1, Industry overview and SWOT. However, specifically regarding technology for zero emission trucks, it is noted that transport policy to date has focussed on emission reduction targets and has remained generally technology neutral.
Methodology
A mixed methods approach has been used to generate the evidence base for this paper. An initial review of published reports and policy positions was supplemented by interviews with representatives of key sectors and a workshop with SMEs and companies working in remote or rural locations that are involved in the freight and logistics sector.
Technology to support the decarbonisation of HGVs
Overview of solutions
The zero-emission truck market is still at an early stage of development and technological solutions for all use cases (range and weight) are not yet available (Decarbonising the Scottish Transport Sector). However, the four main types of vehicle powertrain technologies expected to contribute to the pathway to zero emission trucks are:
- Battery electric trucks (BET)
- Hydrogen based fuel cell electric trucks (FCET), and
- Electric road systems (ERS)
- Low carbon fuels, for example biofuels and electrofuels (e-fuels)
A description of how each of these technologies works is provided in Appendix 1.
In addition to the four technologies identified above, hydrogen Internal Combustion Engine (ICE) and hybrid solutions such as ICE-electric trucks are also available or in development. However, whilst these solutions provide a reduction in emissions, they do not provide technological solutions that are capable of decarbonising to the extent and scale required to achieve Scotland’s 2045 net zero target (HGVs and their role in a future energy system | The ETI). These solutions have therefore not been explored in detail in this Paper.
Comparison of Known Performance
Overview
Figure 1 provides a comparison of the different technologies including vehicle lifecycle GHG emissions, range, speed of charge, cost, energy efficiency, technology readiness and critical dependencies. Internal Combustion Engine Trucks (ICETs) trucks are also included to enable a comparison to existing operations. To demonstrate the different use types, each technology type has been assessed for both “lighter” trucks (predominantly rigid trucks doing urban and regional movements) and “heavier” trucks (predominantly articulated trucks doing long-haul movements). However, it is noted that some lighter trucks are also used for long haul movements and as demonstrated in Paper 1 all types of trucks use urban roads.
It is noted that stated ranges are under test conditions and will not be realised in all real-world conditions. For example, the ranges of battery powered cars and vans have found to be lower than stated in test conditions. The information contained within the Infographic will need to be reviewed regularly due to the fast-moving nature of technology development.
Information used to develop the infographic is summarised in the following sections with a more detailed information in Appendix 2.
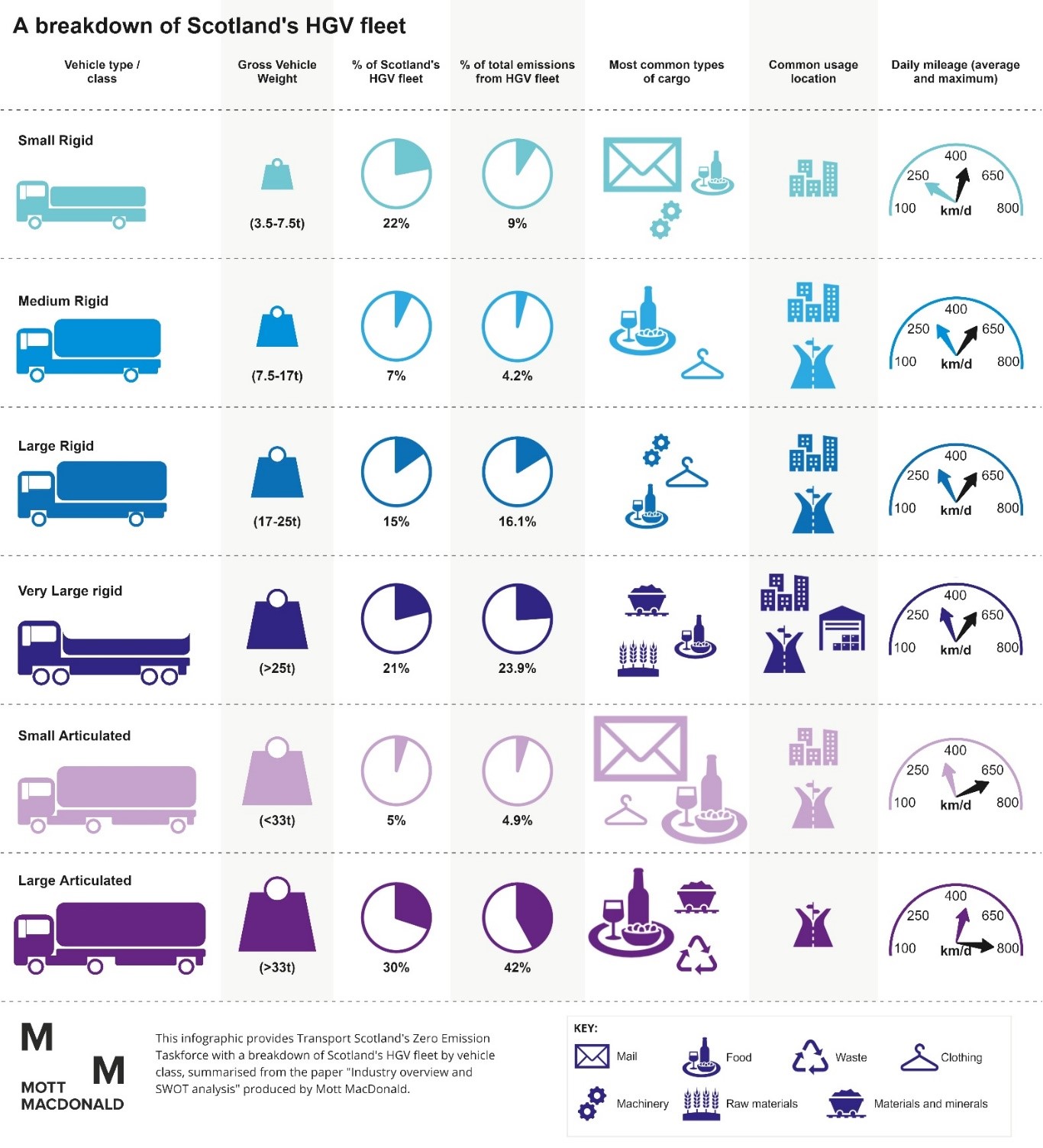
Battery Electric Trucks (BETs)
BETs suitable for short and medium haul trips are currently operating in the UK. For example, Amazon and Tesco recently purchased small numbers of fully electric 37 tonne HGVs. These DAF CF BETs advertise a range of 220 km (depending on the application, driving circumstances and load) and a charge time of 75 minutes.
Environmentally, BET technology is favorable over hydrogen, due to the greater efficiency of direct use of electricity. However, battery technology in commercially available vehicles is currently limited in heavy weight loads and distances of more than around 400 km in one charge. Charge time for larger trucks can also be very long at up to 8 hours for a full charge (using a 150-kw charger - substantially larger chargers are under development). Development challenges include the weight of the large batteries detracting from payload capacity and the long charge times impacting on delivery schedules (Decarbonisation of Heavy Duty Vehicle Transport: Zero emission heavy goods vehicles, Joint Research Centre (JRC), 2021).
Whilst the current market serves short and medium haul trips only, industry developments are fast paced with multiple manufacturers promoting larger BETs with longer ranges and shorter charge times in development. For example, the 40 tonne Telsa Semi (due to be released in 2023) promises a driving range of up to 800 km and a charge time of 30 minutes (with a Megacharger system). Hydrogen range extenders are also in development which may enable BETs to travel up to 750 km with one charge.
However, these longer-range trucks are not yet available to the market resulting in uncertainties regarding their performance. A report completed by the Connected Places Catapult in March 2021 stated that as a stand-alone solution for the largest long-haul HGVs, they do not consider BETs ready for a large-scale demonstration in the short term.
The wide deployment of BETs across all use cases is dependent on significant improvements in battery performance and potentially further amendments to the UK government’s vehicle design standards to allow them to be longer and heavier to support the use of batteries (Decarbonising the Scottish Transport Sector),(Decarbonisation of Heavy Duty Vehicle Transport: Zero emission heavy goods vehicles, Joint Research Centre (JRC), 2021). Critical dependencies also include the installation of sufficient charging infrastructure and grid capacity (discussed in more detail in a future paper).
Fuel Cell Electric Trucks (FCET)
FCETs are not yet being mass produced globally and there have not been any trucks built to UK specifications. However, FCET are currently operating in Europe and Hyundai has delivered 18 tonne rigid FCET trucks in Switzerland in 2020 and has plans to produce as many as 2000 FCET per year for distribution throughout Europe, the US and China.
The technology is well understood in the UK as FCEVs are already used within the bus sector, including in Aberdeen where there are 15 FCEV buses. Scotland will also see more FCEV refuse trucks on the roads in Aberdeen and Glasgow, and FCEV gritters are being explored. Research and development into FCET in the UK context is also being progressed. For example, a trial of Ballard Motive Solutions (previously Arcola Energy)’s 44t truck in Scotland has recently received funding through the DfT’s Zero Emission Road Freight Trials (ZERFT) fund (Zero emission road freight trials funding winners - GOV.UK). Analysis completed for the Committee on Climate Change indicates that FCETs are likely to become more readily available before BET technology can meet the long ranges required.
FCET are expected to be suitable for large, long-haul vehicles as international trials have indicated longer ranges and shorter refueling times than BETs. FCET are reported as currently having an average range of between 350-1250 km per charge and a refueling time of between six and 40 minutes. The range is affected by the volume of hydrogen that can be stored and the efficiency of the fuel cell, which is expected to improve through time (Decarbonisation of Heavy Duty Vehicle Transport: Zero emission heavy goods vehicles, Joint Research Centre (JRC), 2021).
However, FCET are heavily reliant on the availability of green hydrogen production, an extensive network of hydrogen refueling stations and associated infrastructure, which is currently very limited in the UK (this will be the subject of a future paper).
Electric Road Systems (ERS)
ERS technology in the form of a conductive overhead catenary system (similar to that used for electrified rail lines) has been deployed in trials on public roads across Europe and the US where the technology is proven to provide sufficient power for large HGVs at highway speed, albeit over short distances. However, ERS-enabled articulated HGVs are not yet being mass produced.
There are currently no operating ERS trials in the UK, although the DfT has recently provided funding to examine the feasibility for ERS demonstrators in England as part of the ZERFT funding stream (Zero emission road freight trials funding winners - GOV.UK).
The advantage of ERS is the smaller battery requirement, as vehicles can be charged during operation where the infrastructure is available. This results in a longer range than BETs and very minimal (if any) recharge times while operating on the ERS. However, the smaller battery limits the ability of the truck to operate away from the ERS, unless a hybrid technology is used.
Due to infrastructure requirements, ERS is only suited to long-range HGVs and has very high infrastructure development costs, making it potentially uncompetitive with other options. There are also uncertainties around flexibility of use, maintenance costs, transmission loss, practicalities of implementation and the possibility of poorly maintained vehicles disrupting the service.
Low Carbon Fuels (LCFs)
LCFs are fuels which, although not zero emission at the tailpipe, can provide GHG savings compared to fossil fuels on a life-cycle basis. The two largest categories of LCFs relevant to zero emission trucks are biofuels and electro-fuels (e-fuels).
Biofuels are made from biomass (wastes, residues, crops). There is currently growing interest in the use of biomethane as a fuel of high mileage trucks as it can be used in dedicated gas vehicles and has been shown to reduce well to wheel emissions by 85% when compared to diesel.
E-fuels are synthetic fuels that are generated using renewable energy sources. E-fuels are not yet a commercial option, due to high fuel production costs, although trials have demonstrated technological readiness (Roland Berger (2020), Fuel Cells Hydrogen Trucks Heavy Duty's High Performance Green Solution). Their widespread deployment in the HGV sector would rely on the development of large scale green hydrogen production and carbon capture (Analysis to provide costs, efficiencies and roll-out trajectories for zero emission HGVs, buses and coaches. Final report for The Committee on Climate Change, Element Energy 2020) (Roland Berger (2020), Fuel Cells Hydrogen Trucks Heavy Duty's High Performance Green Solution).
Low carbon fuelled trucks are broadly similar to ICETs in terms of range and refuelling times and are currently available and in operation in Scotland. For example, in partnership with IVECO, Glenfiddich converted three CNG (Compressed Natural Gas) powered IVECO Stralis NP 460s trucks into 6x2 44 tonne tractors. Glenfiddich is the first distillery to process all waste on-site, to power its trucks using its own biomethane (produced from distillery by-products) and to have an on-site biogas truck refueling station.
LCFs are potentially an important transition technology to mitigate emissions in the short and medium term, particularly in the 41 tonne plus segment, until zero emission technology has matured. However, there are concerns about the pathway to large scale production of low carbon fuels for HGVs (Shell-Deloitte (2021), Decarbonising Road Freight: GETTING INTO GEAR Industry Perspectives). For example, there are limited biofuel feedstocks available without drawing on food crops and potential competing demand from other hard to decarbonise segments such as aviation and domestic heating. Biomethane also often relies on the existing gas grid for natural gas, which may see changes as we move to net zero.
Total Cost of Ownership (TCO)
Due to the early stage of market development, there is a lack of publicly available data on the costs of zero emission trucks, although all zero emission trucks are more expensive to purchase than equivalent ICE vehicles. Additional information on costs will be presented to the Taskforce as part of a future paper.
Total Cost of Ownership (TCO) includes the following costs in addition to the initial cost of the vehicle:
- Any purchase taxes or incentives
- Cost of borrowing
- Refuelling/recharging
- Maintenance
- Residual value of vehicle or components (eg batteries)
- Any costs associated with end of life (disposal/recycling)
There is a high level of uncertainty around the future TCOs of both zero-emission and ICE vehicles, making comparison difficult. TCO calculations must make assumptions on fuel prices and supply, availability of infrastructure and the take up of different technologies. TCO will also depend on how the HGV is used which adds additional complexity. For example, for small urban delivery vehicles it may be challenging to see return on higher purchase costs because of lower annual mileage, making operating costs highly significant (ULEV Market Segmentation in Scotland).
The uncertainty regarding TCO comparisons is acknowledged in several literature sources which identify the need for greater clarity on the performance and cost of technology to enable industry to have confidence to invest (Shell-Deloitte (2021), Decarbonising Road Freight: GETTING INTO GEAR Industry Perspectives), (ULEV Market Segmentation in Scotland).
Available information indicates that TCO of large rigid BETs and FCET is currently uncompetitive compared to their diesel equivalents and additional measures may be required to encourage a shift to zero emission technologies within the timeframes required (Analysis to provide costs, efficiencies and roll-out trajectories for zero emission HGVs, buses and coaches. Final report for The Committee on Climate Change, Element Energy 2020), (Shell-Deloitte (2021), Decarbonising Road Freight: GETTING INTO GEAR Industry Perspectives). However, studies forecast that TCO will likely be similar or lower than ICE vehicles by 2050 (Final report for The Committee on Climate Change, Element Energy 2020) or sooner (A meta-study of purchase costs for zero-emission trucks. International Council on Clean Transportation (2022)), (Hydrogen Council (2020), Path to hydrogen competitiveness- A cost perspective) (Roland Berger (2020), Fuel Cells Hydrogen Trucks Heavy Duty's High Performance Green Solution), similar to the trajectory taken by car TCO over recent years.
Future technology mix
The immaturity of the ZET markets means there is significant uncertainty and some disagreement around the final plurality of the HGV fuel mix and it is currently unclear which solution (if any) will become dominant. A review of available literature reveals the following:
- It is highly unlikely that a single ZE technology solution will be likely to cover all HGV use cases by 2050
(Decarbonisation of Heavy Duty Vehicle Transport: Zero emission heavy goods vehicles, Joint Research Centre (JRC), 2021) - While there is broad agreement on the potential of different technologies to achieve decarbonisation and the use cases to which they might be best suited, there are different views on how quickly different technologies may enter the market and how widespread their use will be.
- The Dft HGV technology road map assumes a mix of hydrogen and battery electric vehicles from 2040, with the potential for route electrification for some use cases and some routes
- Innovate UK’s Transport Vision 2050 includes a 50/50 electricity/hydrogen mix for HGVs by 2050.
- In the LCF area, biofuels for HGVs are generally treated as a transition technology, partly as biofuels may potentially be required to decarbonise more challenging vehicles used for shipping and aviation, as BET and FCET technology is expected to be available by 2050.
- There are differing views on the use of ERS. The Centre for Sustainable Road Freight White Paper suggests an ERS (catenary system) is the most efficient and cost effective way to decarbonise long-haul HGVs within the timeframe available. However, there are questions regarding the long term business case and the practicalities of installing the infrastructure required.
To achieve the decarbonisation of HGVs by 2050 across the UK, the Connected Places Catapult recommend that key decisions about the mix of technologies are required by 2027 to support the development of a zero-emission marketplace and infrastructure.
Relevance to Scotland
The following section makes observations on how the different technology types may relate to HGVs in Scotland (as presented in Paper 1). This comparison offers the Taskforce some observations which may help inform the development of the pathway to full uptake of ZETs in Scotland.
- Most HGV GHG emissions generated in Scotland are from vehicles for which the technological solution is most uncertain.
- A third of the Scottish HGV fleet is formed by large articulated trucks which are estimated to produce nearly half of the total annual HGV emissions. These vehicles typically deliver food-related products along motorways and have a maximum daily mileage of 600-800km. There are currently no commercially available zero emission models able to meet these requirements. Whilst there are trials being undertaken in the 40-44 tonne range, none are yet able to achieve the long haul distances required.
- Articulated trucks are also the most likely to be impacted by how technology uptake develops in the UK and Europe as these trucks are the main method by which goods are imported and exported between Scotland and the rest of the UK and Europe (see Section 4.1.3 below).
- Very large rigid trucks (over 25 tonnes) are responsible for around 20% of HGV emissions. These trucks are generally used for moving materials from production sites to end users and can have mileage of up to 600 km per day. In addition to technology being currently unavailable at this daily mileage, the goods moved by these trucks are heavy and zero emission technologies may impact on payloads.
There are a significant number of SMEs transporting freight by road in Scotland; 90% of licensed operators have fleets of less than 10 vehicles, 8% have fleets of 10 to 50 vehicles and 2% of companies operate more than 30% of vehicles. Many operators are also located in remote and rural locations. Observations from the research reveal that:
- ERS is not a solution available to all of Scotland. The high cost of the infrastructure means that if installed, it will be on the busiest parts of the trunk road network only. In addition, road freight is a reserved matter (see Background Paper 1, Industry overview and SWOT) which may limit the ability of Scotland to influence the implementation of this technology.
- BET may seem less risky for smaller operators as charging infrastructure may be easier to access than hydrogen. Conversely, FCET may be more attractive to smaller operators due to longer ranges and the ability to rely on public refuelling infrastructure as opposed to taking on the cost of installing private BET charging infrastructure themselves.
- The lack of TCO information is likely to disproportionately impact small operators. Larger operators will likely have access to more information on trials and vehicles operating longer mileage are less sensitive to the costs of different fuel types.
Scotland’s pathway to zero emission trucks will be influenced by internal and external factors:
- Around 40% of Scotland’s freight is transported out of Scotland, mostly to northern parts of England. Interoperability and alignment between borders with other parts of the UK is therefore a critical consideration. For example, if the UK government was to adopt an ERS, this may significantly alter business cases within Scottish fleets.
- Whilst less than one million tonnes of road freight is transported from Scotland to outside of the UK, the technological solutions adopted and developed in other countries will influence the market in terms of the range of solutions and ZET models available to the Scottish and UK market. This is of particular importance as the longer range vehicles produce the most emissions and have the most uncertainties regarding a technological solution.
- The decarbonisation pathway of other transport modes may also influence the optimal outcome for HGVs. For example, if the marine sector decided to adopt hydrogen as a dominant fuel, this would increase the availability of hydrogen at ports, resulting in easier access for HGVs.
Appendix 1 – Description of technologies and case studies
Battery Electric Trucks (BETs)
Battery Electric Trucks (BETs) are propelled by electric motors using energy stored within onboard batteries. The primary components of a BET are described and shown below. (Electric Cars Report (2013). Brusa to Supply Drivetrain Components for E-FORCE Electric Truck)
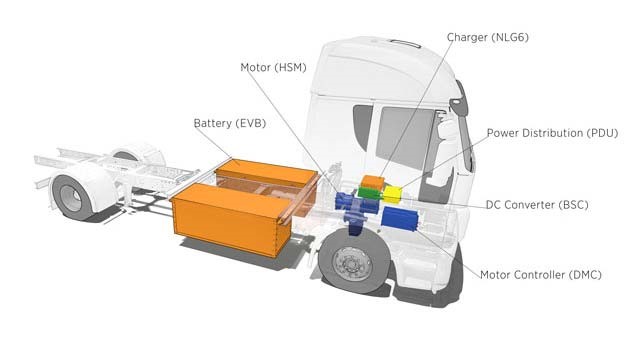
- Charger – a device that charges the battery and converts Alternating Current (AC) electricity to Direct Current (DC) electricity
- Battery – stores electrical energy in the form of DC electricity
- Power distribution – converts DC from battery to AC which is used by the motor
- Motor controller – regulates electrical energy from battery based on voltage required
- Motor – takes electrical power from controller to turn the transmission and wheels
- There are several different technological approaches for BET, as follows:
- Pure BET: Electric motors installed within each axel, with batteries integrated within the chassis for optimum weight distribution and power delivery. These chassis are designed to be electric only with no Internal Combustion Engine (ICE) options. Typically, a pure BET chassis can accommodate more battery capacity resulting in a larger range.
- Mixed powertrain chassis: These are designed by the manufacturer to either be fitted with an ICE with fuel tanks or with electric motors and batteries. In this instance, the electric motor(s) replace the space occupied by the ICE with batteries mounted in locations designed to store batteries.
- Chassis conversion: Existing ICE HGV chassis converted to a BET. Electric motor(s) replace the ICE, and batteries are fitted where space permits. As there are no locations specifically designed to store batteries, the overall battery capacity (and range) is lower.
BET Case Study: Leyland’s DAF Electric Truck Trial
Location
United Kingdom
Description of the Trial
Leyland Trucks has been selected to support the UK Government’s Department for Transport (DfT) efforts to encourage the use of battery electric vehicles in commercial transport. Its trucks are to participate in a project designed to help UK operators make the transition to battery electric trucks.
A total of 20 DAF LF Electric 19-tonne rigids, together with the required charging infrastructure, will be fitted with data logging equipment and used to support the UK Government’s Department for Transport (DfT) efforts to encourage the use of battery electric vehicles in commercial transport. An interactive website will inform future fleet operator buying decisions and help stimulate the sale of battery electric trucks. The project is scheduled to commence this summer.
To support operators in understanding the costs and considerations around the deployment of zero emission trucks, the DfT is adopting a proactive campaign to assist the road transport sector in its transition. The project is part of the Government’s £20 million zero emission road freight trials.
The project is delivered using the SBRI (Small Business Research Initiative), which is a process that brings together government challenges and ideas from business to create innovative solutions. Leyland Trucks will work with CENEX, the low carbon research consultancy, to create an interactive report and website using real-world data to assist public and private fleet operators to better understand the strengths, challenges, and deployment options around zero emissions trucks.
Outcomes
- Leyland Trucks manufactures the successful DAF LF series of distribution vehicles, including the LF Electric which recently entered production at its state-of-the-art plant in Lancashire.
- Leyland Trucks will be partnering several end-users in the public sector during the trial, including various NHS (National Health Service) operations around the country earmarked to trial eleven DAF LF Electric rigids. The remaining nine vehicles will go to a number of Local Authorities to support recycling and deliveries to schools. Bodywork will include ex-factory PACCAR box bodies and refrigerated bodywork from Gray & Adams.
(Source: leylandtrucksltd.co.uk)
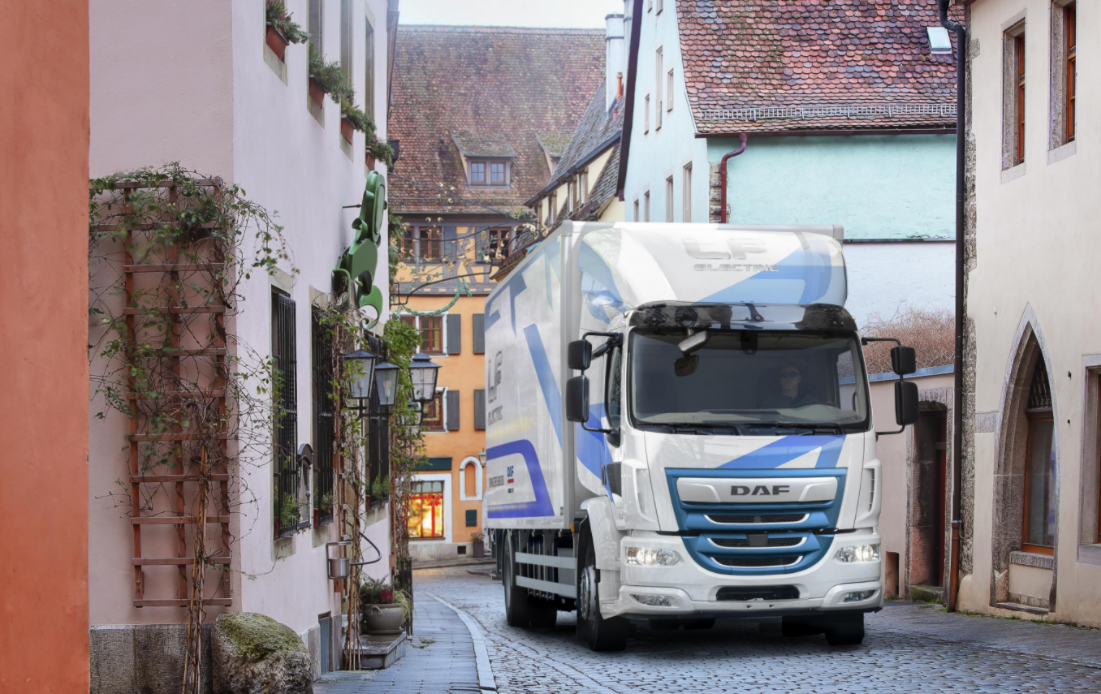
Fuel Cell Electric Trucks (FCET)
Hydrogen Fuel Cell Electric Trucks (FCETs) have similar characteristics to BET powertrains, both utilise electricity to power electric motors for propulsion. The major distinction between each lies in the source of the energy used to produce the electrical charge which in turn powers the motors. Whilst BEVs depend on electrical fuelling drawn upon directly, the major feature distinguishing FCEV’s is that they use a fuel cell which act as a miniature powerplant onboard the vehicle. This means that the energy conversion occurs within the vehicle during its movement, not at some point prior to journeying.
The primary components of a FCET are described and shown below:
- Hydrogen tanks – stores hydrogen within the vehicle that is then pumped to the fuel cell
- Fuel cell – a chemical reaction from hydrogen and oxygen within the fuel cell extracts electrons from the hydrogen and creates electricity
- Battery pack – charged by the fuel cell reaction
- Electric powertrain – powers the vehicle using electricity generated by fuel cells
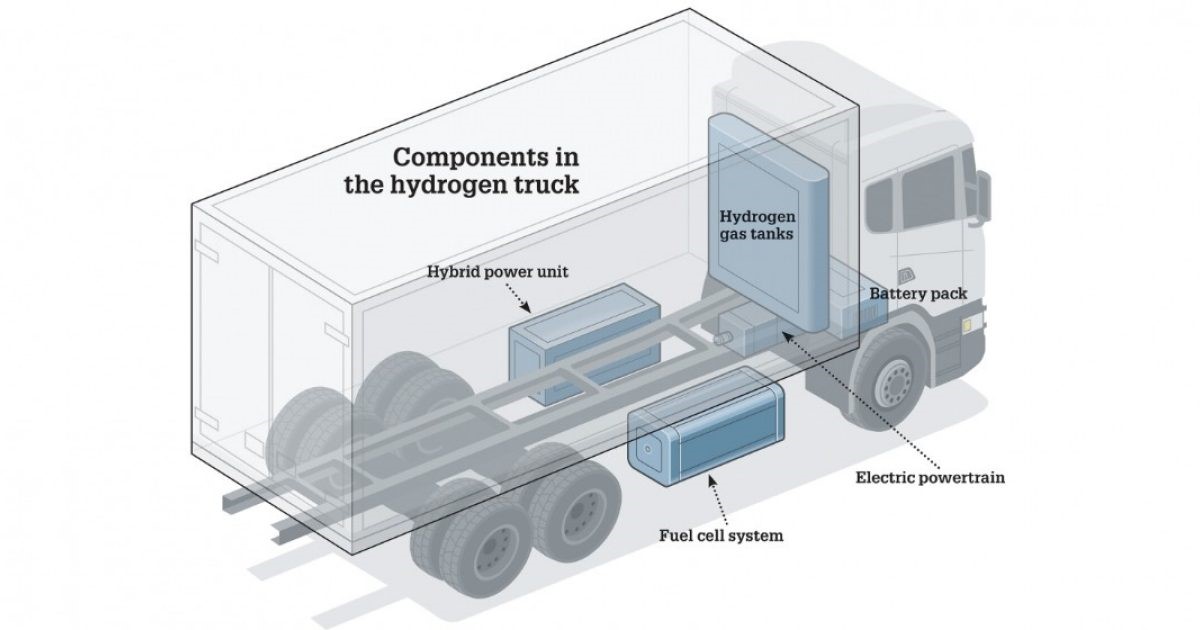
Catenary Electric Trucks (CETs)
Catenary Electric Trucks (CET) operate on eHighways or Electric Road System (ERS) and use electrical power from overhead catenary lines which removes the need to store as much energy on board. CETs can be powered by pure-electric if they are on an ERS, but hybrid models increase flexibility by enabling travel beyond the ERS. Hybrid models tend to be either ICE (in the short term noting this technology would not meet net zero requirements) or BEV.
The key components of a CET are shown and described below:
- Pantograph – power receiver device that connects to overhead catenary lines to power vehicle, follows and automatically detaches from catenary
- dc/dc convertor – converts catenary line power to truck voltage level
- Battery – used to store energy from catenary and regenerative braking and to power vehicle when not on ERS
- E-motor – uses electricity from catenary and battery to power vehicle
- Fuel tank – in ICE hybrid models fuel tank can store fossil fuels or biofuels
- Combustion engine – in hybrid ICE models powers vehicle when not connected to ERS
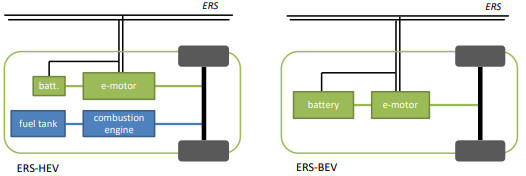
The introduction of ERS and CETs would require a coordinated and often national effort. Despite being applicable to other use cases, the aim of using ERS to electrify long-haul freight is what has pushed forward the development and trialling of the technology.
ERS Case Study: Scania Trucks with CET Technology
Location
Germany
Description of the Trial
The first German ERS track on the A5 motorway near Frankfurt will be extended by almost 7km, and Scania will deliver seven additional pantograph-equipped trucks to operate on this expanded stretch of electrified motorway.
Three trials with electrified trucks are ongoing in Germany. Two types of Scania truck with different strong electrified powertrains are being tested in the demonstration project. In the latest expansion, an additional 7km of overhead catenary lines will be added to the A5 motorway segment in Hessen, bringing the total to 12km in the direction of Darmstadt and 5km towards Frankfurt. This work is scheduled to be completed by the end of 2022. With 135,000 vehicles daily, including 14,000 trucks, the A5 motorway south of Frankfurt is one of the busiest and most heavily polluted sections of road in Germany.
The A5 is one of three catenary lines being conducted in Germany. In late 2019, an additional segment of electrified road was opened near Lübeck in Schleswig-Holstein (A1) where one Scania truck is currently being tested. A third trial will start in Baden-Württemberg (B462) in 2021. In total, 22 trucks will be in operation on these three test tracks.
Outcomes
- The hybrid drivetrain enables the optimization of carbon emissions reduction, costs and weight.
- This flexibility reduces the negative impact on the customers’ logistics and allows trucks to drive in electric mode in urban areas.
- In the future, with more catenary infrastructure, a pure battery truck can be the base vehicle for this technology.
- Electric roads offer rational and effective charging en route. While this is technically just another way to charge electric trucks, the solution can be particularly relevant on heavily trafficked roads, and additionally it saves batteries and reduces load on the energy network.
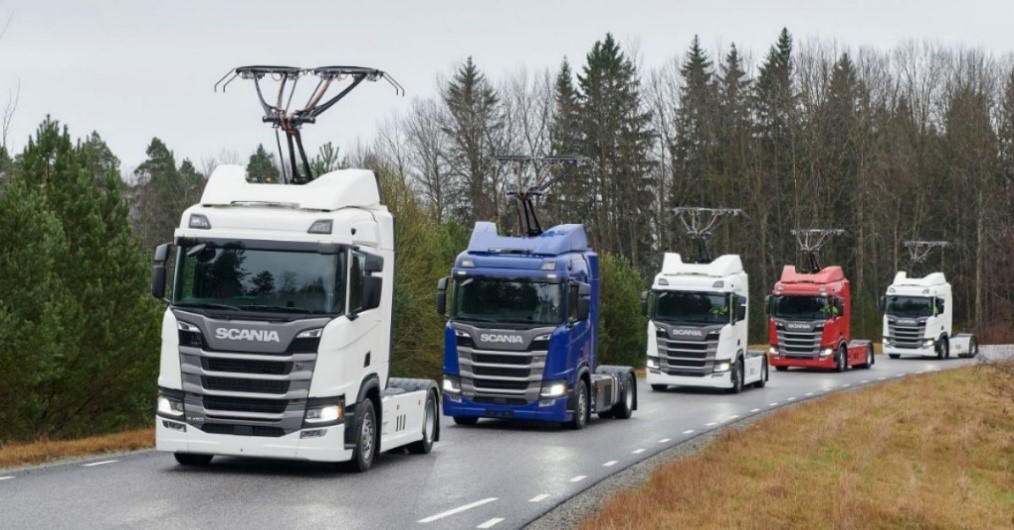
Low Carbon Fuels
LCFs are fuels which, although not zero emission at the tailpipe, can provide GHG savings compared to fossil fuels on a life-cycle basis. The two most relevant categories of LCFs to a zero emission truck pathway are biofuels and electro-fuels (e-fuels).
Biofuels are made from biomass (wastes, residues, crops). Biofuels are not carbon dioxide free, however the carbon dioxide emitted is derived from the absorption of atmospheric CO2 by the plant for photosynthesis. The fuels can be categorised as coming from more or less ‘sustainable’ sources, depending on the wider carbon impacts of their cultivation and use.
E-fuels are synthetic fuels that are generated using renewable energy sources. Hydrogen is first produced, typically using electrolysis in the same way as for FCETs (In the revision to its Renewable Energy Directive (RED II), the EU defines hydrogen and e-fuels produced in this way as renewable fuels of non-biological origin (RFNBO). Some studies also classify hydrogen as an e-fuel). This can be synthesised into other fuels using other carbon-based feedstocks, including carbon dioxide. Carbon dioxide is available from many sources such waste gas from industrial processes, biogenic CO2 from bakeries, ethanol fermentation in breweries or ethanol fuel plants, as well as from ambient air capture (Roland Berger (2020), Fuel Cells Hydrogen Trucks Heavy Duty's High Performance Green Solution), (Renewable fuels of non-biological origin (RFNBO) (etipbioenergy.eu)). Electro fuels are not yet a commercial option, but the development of large scale green hydrogen production and carbon capture may see them become a significant future fuel in at least one of the transport sectors.
For performance improvements, it is possible to mix some LCFs with traditional fuels to find a balance between appropriate performance with some emission reduction. However, higher levels of blending involving more fossil fuels would increase GHG emissions.
Biofuels Case Study: Glenfiddich Delivery Trucks
Location
North-eastern Scotland
Description of the Trial
During the whisky making process there is a significant amount of byproduct that can be used to create a circular economy. William Grant & Sons, the independent owner of Glenfiddich Distillery located in Dufftown in north-east Scotland, has created a “Fueled by Glenfiddich” initiative to decarbonise its HGV fleet by producing biomethane by anaerobically digesting the waste they generate.
In partnership with IVECO, Glenfiddich converted three CNG Compressed Natural Gas-powered IVECO Stralis NP 460s trucks into 6x2 tractors with a GVW of 44-tons. Glenfiddich is the first distillery to process all waste on-site, to power its trucks using its own biomethane and to have an on-site biogas truck refueling station.
Outcomes
- Conversion of IVECO Natural Power trucks to carry more weight (44tn).
- Biogas trucks used to transport Glenfiddich whisky from production in Speyside to bottling and packaging in central and western Scotland.
- Use of biogas cuts CO2 emissions by over 95% compared to diesel and other fossil fuels.
- Reduction of other harmful particulates and GHG emissions by up to 99%.
- Each truck will displace up to 250 tons of CO2 annually.
- Could be scaled up to Glenfiddich’s own fleet of approx. 20 trucks, to other William Grant & Sons owned distilleries and to fuel other company’s trucks.
- Supporting the Scottish whisky industries aims to hit net zero targets by 2040.
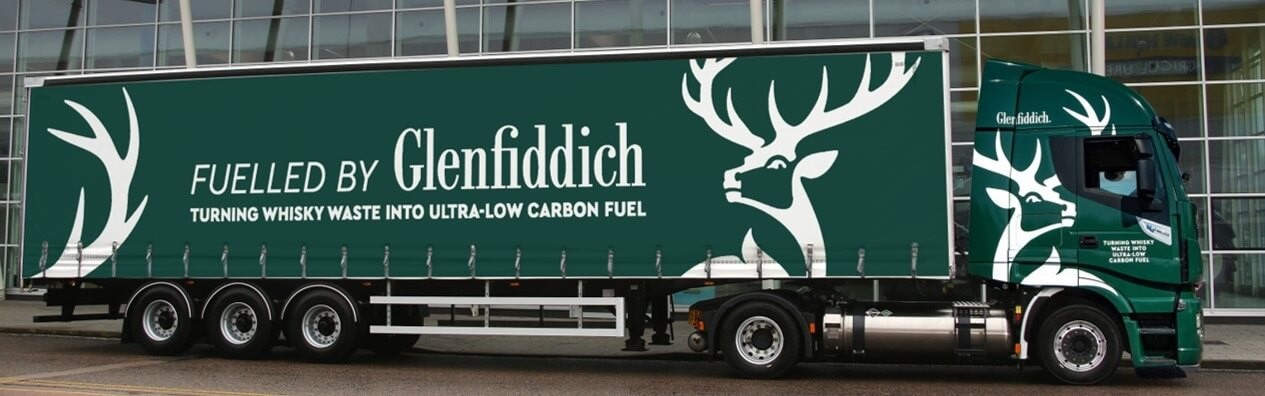
Appendix 2 – Advantages and disadvantages of technologies
BET pros
- Currently available and in operation for urban delivery and other short haul trips.
- Opportunity to align charging with use-patterns including overnight and strategic motorway charge points (eg at 50km intervals).
- High powered chargers in development and expected by 2023. Truck manufacturers are pushing for the construction of a megawatt charger network in Europe and potential locations for fast chargers have been proposed.
- ICE models can be converted to BET in some cases. (Though BVRLA report the consensus is that retrofit options will not be cost effective for most truck operators)
- Best applied to short range logistics and specific applications as current technology stands.
- Lower operating costs vs ICE.
- TCO expected to reduce to be more in line with ICE vehicles over time (on a similar pathway to cars).
- Lower GHG emissions throughout lifecycle than other technology types (bar CETs).
- Energy efficiency three times greater than ICE and twice than hydrogen.
BET Cons
- Heavy duty and long-haul trucks require high energy consumption to charge.
- Existing BETs available max range 400 km.
- High power chargers required for short charging times – up to 1.2MW. Likely need for grid upgrades.
- The heavier long-haul trucks require large batteries that take up payload, particularly on articulated trucks.
- Recharging time may result in missing mileage.
- Heavy batteries may result other components being heavier too.
- Costs, scarcity and volatility of supply of raw materials for batteries may affect cost and availability of required battery volumes for required power demand across transport sector.
- Operational differences compared to ICETs may lead to need for more vehicles in fleets or other accommodations.
- High upfront cost of purchasing vehicle.
- Resale value of vehicle and component parts such as battery is an unknown/concern for operators.
- High dependency on charging infrastructure, including on route for longer journeys.
FCET Pros
- Versatile technology applicable to a wide range of use cases
- Well suited to back-to-base refuelling
- Similar pattern of use to ICETs so unlikely to disrupt operational efficiency
- Greater operating ranges compared to BETs
- Cost will reduce as use of hydrogen expands
- Storage tanks take up less space than battery packs for similar range
- Only water emitted from truck
FCET Cons
- Expensive upfront costs compared to ICE.
- Limited range of manufacturers and therefore high production costs.
- Unclear TCO, particularly whether higher or lower than BETs.
- High utilisation of infrastructure required to ensure it is cost efficient.
- Availability of hydrogen dependent on wider economy.
- Low energy efficiency compared to other solutions – on a well-to-wheel basis hydrogen is 4-6 times less efficient than BET.
- Hydrogen storage requires expensive materials.
- Hydrogen storage takes up space compared to ICE.
- Component electrification and thermal management present further challenge.
- Operators have some concerns about Fuel cell durability due to lack of available information.
- Zero carbon credentials depend on source of hydrogen.
- Not yet accepted standards for refuelling infrastructure between OEMs.
- High dependency on the availability of refuelling infrastructure.
- Cost of hydrogen may vary depending on location.
ERS Pros
- Catenary lines and pantographs have been well-tested on the railways.
- Has seen significant trials in Germany and Sweden (Siemens eHighway).
- CET vehicles have been designed and trialled.
- Lower energy costs compared to ICE.
- Suitable for high frequency point-to-point routes (i.e., strategic motorways)
- Centre for Sustainable Road Freight argue (The Centre for Sustainable Road Freight (SRF) (2020), Decarbonising the UK’s Long-Haul Road Freight at Minimum Economic Cost):
- Converting 7500 km of the UK road network could serve 65% of UK road freight trips. The proposed network includes the Scottish central belt, the A9 as far as Inverness and the A92 to Aberdeen.
- Fleet owner could get payback from investment in CET after 18 months (due to lower energy costs).
- Energy efficiency of 80-85% from substation to wheel (assuming vehicles use the ERS only).
- Regenerative braking can recharge battery or go back into catenary.
- less requirement for recharge/refuel breaks when operating on the ERS.
- Can extend range through use of hybrid technology (range varies depending on technology used).
- Low operational costs for operators, comparable to BETs but without need for large battery storage (for trips using the ERS).
ERS Cons
- HGV operational model and vehicles starkly different to heavily regulated rail sector.
- Requires complex co-ordination between infrastructure and compatible fleet of vehicles.
- May only reach key points on strategic road network, eg cities. Requirement for potentially substantial distances to be travelled off the ERS system, especially to remote and rural areas. Requirement for large battery/ other propulsion may impact on payload and reduce stated benefits associated with smaller battery.
- Stated benefits likely to be significantly reduced in Scotland due to high number of operators in remote and rural locations.
- Would have to be implemented across UK and potentially wider.
- Less flexible than other solutions.
- High upfront investment required for both infrastructure and vehicles.
- Ongoing maintenance of infrastructure.
- Health and safety concerns relating to level of voltage.
- Entirely dependent on adequate infrastructure – completely unrelated to fleet owners.
- Potential objections to the installation of the infrastructure.
- Pantograph potentially causes aerodynamic drag.
- Loss of energy between grid and pantograph.
- Concept not well known or understood within Scottish market.
- ICE hybrid CET unlikely to meet Scottish GHG mission reduction targets without offsetting.
Low Carbon Fuels Pros
- Biofuels relatively well established. Can be implemented now and provide significant GHG emission reductions.
- Close to ‘drop in ‘ replacement.
- Opportunity to transition to lower emission fleet whilst other technologies are still in development.
- Low fuel duty rates for natural gas used in transport (including biomethane).
- Biomethane can fulfil a niche option for decarbonising freight (and other transport applications / sub-sectors).
- E-fuels could potentially be used as a drop in replacement for diesel and use existing infrastructure.
Low Carbon fuels Cons
- Limited availability of biofuel feedstocks
- E-fuels are currently not commercially viable due to very high production costs
- May be unattractive to some operators who may prefer to opt for a zero-emission technology to avoid stranded assets
- May be competition for the use of low carbon fuels with other transport modes such as aviation, where zero emission technology is further behind HGVs
- Can only be a bridging solution until zero emission technology matures. Not zero emission at the tailpipe.